INTRODUCTION
In mammography, compression of the breast is essential to generate images with diagnostic quality. Besides allowing reduction in the radiation dose, compression prevents the patient motion during exposure and aids in the breast tissues distribution, reducing tissues overlapping and facilitating the visualization of breast structures on images(1—3).
Currently, different methods are suggested to perform tests for evaluating the mammographic compression force(4—6), in addition to the development of specific dynamometers for such testing(3). The present study was developed to evaluate the intermethod agreement in the measurement of compression force as well as variations in the testing of different mammography apparatuses.
MATERIALS AND METHODS
The present study involved the undertaking of tests suggested by the Radiodiagnosis Guidelines mentioned on the Resolution RE 1016 of Agência Nacional de Vigilância Sanitária (Anvisa) (the Brazilian Health Surveillance Agency)(4). Furthermore, the methodologies proposed by the European Guidelines for Quality Assurance in breast cancer screening and diagnosis(5) and American Association of Physicists in Medicine(6) were employed. Different force measurement devices (dynamometers) were utilized, namely, one Keithley dynamometer, one Sunrise standard model, and one RMI 163 model specific for mammography, besides one KRATOS extension dynamometer (testing range up to 100 kgf), always attached to the compression paddle at 2—5 cm from the chest wall. Additionally, different materials were utilized to offer resistance to compression: tennis balls (as recommended by the European Protocol), plastic balls (Killp Plásticos Ltda) and hospital gauze cloths.
The tests were accomplished on the following mammography units: Siemens Mammomat 3000, Philips Mammo Diagnostic UC and GE Alpha ST.
In the testing with the RMI instrument, the data collection was made in different ways, as follows: with the compression paddle over the dynamometer support plate; with one tennis ball or plastic ball placed between the compression paddle and the support plate; and the hospital gauze cloth folded in 2, 4 and 16 parts, placed between the compression paddle and the support plate. On the other hand, with the bathroom-scale-type dynamometer, the data collection was made as follows: either with one or with two tennis/plastic ball(s) placed between the compression paddle and the surface of the scale support plate; and with the hospital gauze cloth folded in 2, 4 and 16 parts and placed between the surface of the scale support plate and the compression paddle. The extension dynamometer was utilized between the X ray tube and the compression paddle. Figure 1 illustrates the geometry of some of such tests.
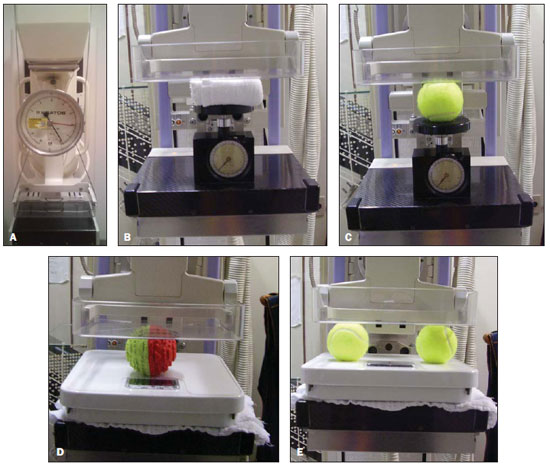
Figure 1. Photos demonstrating the data collection with a KRATOS dynamometer (A), RMI dynamometer with the hospital gauze cloth folded in 16 parts (B), placed between the instrument support plate and the compression paddle and one tennis ball (C), and Keithley dynamometer with a plastic ball (D) and with two tennis balls (E) between the scale support plate and the compression paddle.
The measurements performed with the extension dynamometer and according to the European Protocol, were established as a comparative reference among the different testing methods. Thus, the different measurement methods were evaluated and the respective results were compared for the different mammography units, measurement devices and resistance objects. Table 1 reflects an overview of the data collection.
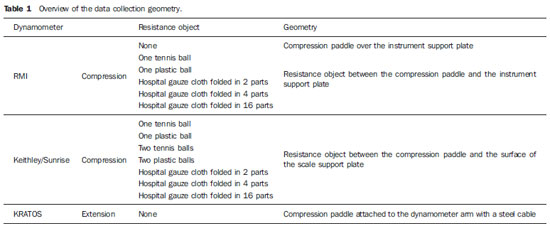
Ten measurements were performed for each data collection geometry. Besides the mean value of maximum compression force of the unit, the existence of more than one compression stage was evaluated. A 18 cm × 24 cm bucky device was considered for the purpose of the mammography units comparison. Additional measurements were performed with the Mammomat 3000 unit utilizing a 24 cm × 30 cm bucky, and no significant difference was observed with the change in the size of the compression paddle. The Keithley and Sunrise dynamometers did not present any significant difference (variations < 5% in the mean values) among measurements and in the presence of a second compression stage. For this reason, the authors have opted to report the results achieved with the Keithley instrument.
With this methodological proposal, the European(5), American(6) and Brazilian(4) protocols recommendations could be compared. Also, it was possible to evaluate the differences between the results with the different resistance objects utilized to simulate the breast under compression. Additionally, the dependence of the resulting compression force over the resistance object material was evaluated.
RESULTS
The results of the present study are presented on a sequence of graphics with mean value and standard deviation for measurements of compression force performed at the first and second stages of compression. Figure 2 shows graphics with the results observed for each equipment and method evaluated.
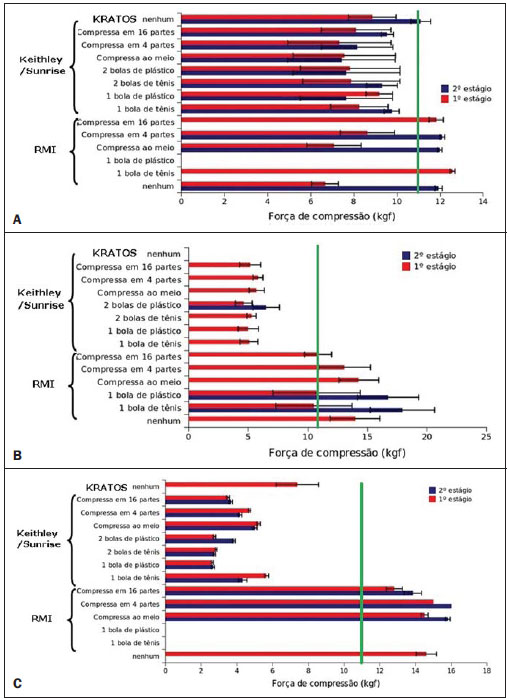
Figure 2. Graphics demonstrating mean compression force values for each method and its respective standard deviations, at the presented compression stages, considering the Siemens Mammomat 3000 (A), Philips Mammo Diagnostic UC (B) and GE Alpha ST (C) apparatuses. The green solid line represents the minimum compression force threshold (11 kgf).
The absence of compression force data regarding any equipment (i.e., no information on the respective column on the graphics included in Figure 2), corresponds to a test that has not performed because of any technical limitation of the method or the equipment itself, as discussed below.
All the tested units had their respective reports forwarded to the partner institutions for the necessary corrections.
DISCUSSION
Amongst the tested mammography units, the Mammomat 3000 apparatus presented the highest number of secondary compression stages (85%). Because of technical limitations regarding positioning of the compression paddle and height of the RMI instrument, the test with the plastic ball could not be performed in this equipment whose compression force values have always remained below the maximum normative threshold of 18 kgf.
The measurements with the Keithley (with no resistance object), RMI and KRATOS instruments presented mean values between 11 and 13 kgf. The Keithley dynamometer presented the smallest variation in results as the measurement methods evaluated in the present study are compared.
On the other hand, the Mammo Diagnostic UC apparatus presented compression stability, with the smallest number of second compression stages (23%). Such equipment did not allow the utilization of the extension dynamometer. Also, it was the only one that presented compression force values close to the maximum Brazilian normative threshold (18 kgf) in the testing with a second compression stage, with and without resistance objects, utilizing the RMI dynamometer. However, the standard deviations calculated for each of the measurements demonstrated values above such threshold. The Mammo Diagnostic UC equipment was the only one that did not present secondary stages as resistance objects were added. In the measurement method with the Keithley dynamometer, the values were always below the minimum normative threshold. The testing with the extension dynamometer could not be performed because of the equipment limitation but at the technical lead request.
The GE Alpha ST equipment did not allow the measurement with resistance objects and the RMI instrument because of the unit limitations. All the compression force measurements resulted in values below the maximum threshold established by the Brazilian standards. On the other hand, the measurements performed with the RMI and KRATOS dynamometers remained between 11 kgf and 17 kgf, i.e., within the compression force threshold established by the Brazilian standards.
As regards the methods comparison, it may be said that the smallest variations in the measurements with the extension dynamometer were observed amongst the measurements performed with the RMI instrument. On the other hand, the greatest variations were observed in the measurements with the bathroom scale-type dynamometer, probably because the surface of such instrument is larger than the area covered by the breast and many times forces the support plate, leading the compression system to “perceive” the resistance object in a different way and, consequently, resulting in underestimation of the compression force value.
It is known that the utilization of compression force greater than the Brazilian normative threshold may result in damages to the breast tissue. On the other hand, compression force smaller than the one established by the Brazilian standards may impair the visualization of relevant structures in the breast cancer diagnosis.
The dynamometers utilized in the present study were calibrated and presented constancy in a standard measurement environment. Thus, the variations observed amongst the ten measurements with a same dynamometer must be due to instability in the compression system of each mammography unit. The differences observed amongst the mean compression force values for a same equipment may be caused by the proposed method (particularly by the inclusion of a resistance object to simulate the breast) and by the compression system of the equipment. Overall, the apparatuses presented smaller percentage variations in the measurements at second compression stages, as applicable.
It is important to observe that resistance objects such as tennis balls and plastic balls may present variable features over time, which may change the test response as such objects are utilized.
The intermethod differences reinforce the importance of utilizing a method that results in more accurate and precise measurement of breast compression force, considering that this is essential to generate mammographic images with diagnostic quality.
CONCLUSIONS
Based on the results of the present study, it may be observed that there are significant differences among the applied methods, probably because of differences in the compression systems of each mammography unit.
The compression test result depends on both the testing method and the compression system of the mammographic equipment. The smallest variations were observed with the extension dynamometer, and the greatest ones, with the bathroom scale-type dynamometer (underestimated compression force values).
Resistance objects allow a better simulation of breast compression and, for this reason, should be utilized in cases where compression dynamometers are utilized as measurement instruments. However, it is recommended that the resistance objects (tennis and plastic balls) use time is observed, considering that their resistance force decreases with use.
Considering the present results, it is suggested that compression force measurements are performed with extension dynamometers, provided they are fixed to the compression paddle, in the region at 2-5 cm from the chest wall. Such location is the best to simulate the breast reaction to compression, presenting smaller variation in data (good results reproducibility). Among the proposed methods, this is the one that is less influenced the mammography equipment compression system.
It is important to observe that significant differences were observed among compression systems and among proposed methods. Thus the choice of the way the compression test is performed is critical for the acceptance of the mammography equipment, the technical lead being the responsible who will determine the most appropriate and realistic testing method.
REFERENCES
1. Hoff G. Cálculo da dose em glândula mamária, utilizando o código de transporte de Monte Carlo MCNP, para as energias utilizadas em mamografia [dissertação]. Rio de Janeiro, RJ: Universidade do Estado do Rio de Janeiro; 2005.
2. Rothenberg LN. Exposures and doses in mammography. In: Haus AG, Yaffe MJ, editors. Syllabus: a categorical course in physics — technical aspects of breast imaging. 3rd ed. Chicago, IL: Radiological Society of North America; 1994. p. 113—20.
3. Godinho ER, Koch HA. Rastreamento do câncer de mama: aspectos relacionados ao médico. Radiol Bras. 2004;37:91—9.
4. Brasil. Ministério da Saúde. Agência Nacional de Vigilância Sanitária. Radiodiagnóstico médico: desempenho de equipamentos e segurança. Série A. Normas e manuais técnicos. Brasília, DF: Ministério da Saúde; 2005.
5. Perry N, Broeders M, de Wolf C, et al. European guidelines for quality assurance in breast cancer screening and diagnosis. 4th ed. Luxembourg: Office for Official Publications of the European Communities; 2006.
6. Butler PF. Changes in the 1999 ACR mammography quality control manual. In: Haus AG, Yaffe MJ, editors. Categorical course in diagnostic radiology physics: physical aspects of breast imaging current and future considerations. Oak Brook, IL: Radiological Society of North America; 1999. p. 123—99.
1. Specialist in Radiodiagnostic Physics – Associação Brasileira de Física Médica (ABFM), Managing Partner of AFIM Assessoria em Física Médica Ltda, Porto Alegre, RS, Brazil.
2. Graduate Student of Medical Physics, Trainee at AFIM Assessoria em Física Médica Ltda, Porto Alegre, RS, Brazil.
3. Supervisor of Radiological Protection in Nuclear Medicine – Comissão Nacional de Energia Nuclear (CNEN), Managing Partner of AFIM Assessoria em Física Médica Ltda, Porto Alegre, RS, Brazil.
4. Post-graduate of Projects Management, Managing Partner of AFIM Assessoria em Física Médica Ltda, Porto Alegre, RS, Brazil.
5. PhD, Professor TI 40, School of Physics – Pontifícia Universidade Católica do Rio Grande do Sul (PUCRS), Porto Alegre, RS, Brazil.
Mailing Address:
Dra. Gabriela Hoff
Avenida Ipiranga, 6681, P10/sala 207, Partenon
Porto Alegre, RS, Brazil, 90619-900
E-mail: ghoff.gesic@gmail.com
Received September 4, 2010.
Accepted after revision April 27, 2011.
Study developed at AFIM Assessoria em Física Médica Ltda and by the Group of Experimentation and Simulation in Medical Physics of Pontifícia Universidade Católica do Rio Grande do Sul (PUCRS), Porto Alegre, RS, Brazil.